MIT Develop Resilient Polymer Muscles on Mini Drone
Breakthrough made for tougher drone design.
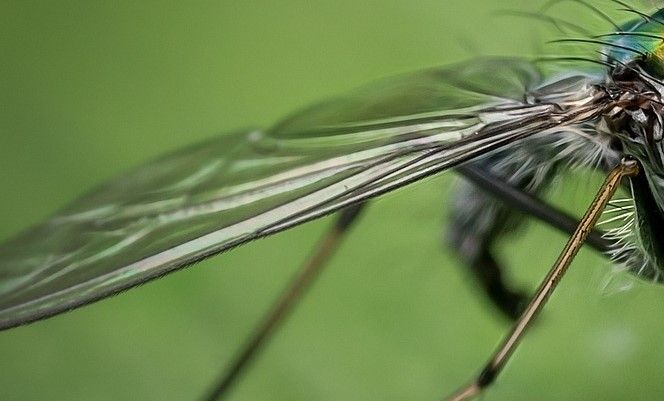
Anyone who has ever seen a fly attempting to leave through a window, knows that they are not the most accurate fliers. The same can be said for other insects such as bumblebees, mosquitoes, and butterflies.
Yet while they are always crashing into things (or each other) they never seem to get very damaged – at least not enough to stop them flying.
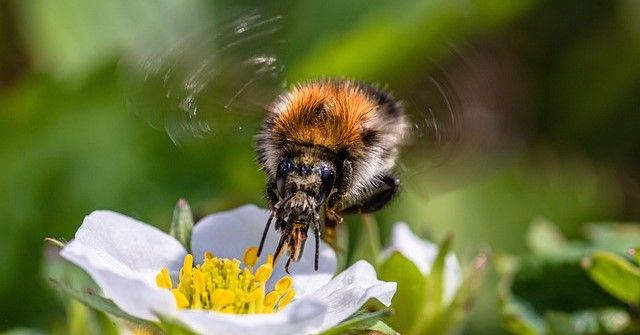
Drones, on the other hand, are not so sturdy. The slightest contact with an object can send them crashing to the ground – often permanently.
Researchers from MIT have now come a long way to solving the fragility of drones with their own insect-inspired robot which has “resilient artificial muscles that can … effectively recover flight performance after suffering severe damage.”
It is an application of polymer know-how and carbon nanotubes that could revolutionise next-generation drone design. Enabling damaged parts to be isolated to maintain flight and for broken parts to even be repaired with a novel laser technique.
As the MIT website reports, “Using [the new] techniques, a damaged robot could maintain flight-level performance after one of its artificial muscles was jabbed by 10 needles, and the actuator was still able to operate after a large hole was burnt into it. [While] repair methods enabled a robot to keep flying even after the researchers cut off 20 percent of its wing tip.”
This amount of damage would have grounded conventional-design drones despite recent improvements in flying capabilities. As the study, now published in the journal Science Robotics, states, “Although robots driven by rigid actuators have demonstrated agile locomotion and manipulation, most of them lack animal-like robustness against unexpected damage.”
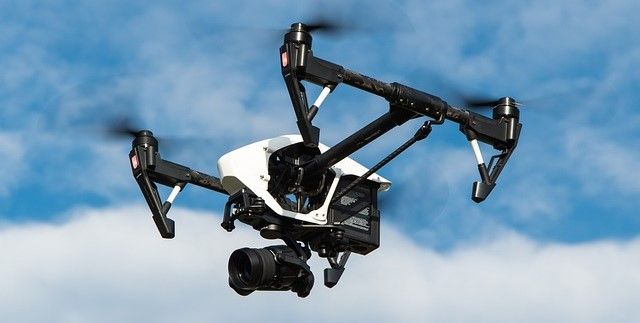
“We spent a lot of time understanding the dynamics of soft, artificial muscles and, through both a new fabrication method and a new understanding, we can show a level of resilience to damage that is comparable to insects,” explains Kevin Chen, a Jr. Assistant Professor at the Department of Electrical Engineering and Computer Science (EECS) and the paper’s senior author. “We’re very excited about this. But the insects are still superior to us, in the sense that they can lose up to 40 percent of their wing and still fly. We still have some catch-up work to do.”
The tiny, rectangular robots being produced in Chen's lab are about as long as a AA battery and twice as wide, yet weigh no more than a paper clip. Dielectric elastomer actuators (DEAs), which are soft artificial muscles, are used to power the wings on each corner. These muscles are made of elastomer layers which are placed between two incredibly thin electrodes and wrapped into a pliable tube. The electrodes pressure the elastomer when voltage is provided to the DEA - causing the wing to flap.
It is an incredible design and a leap forward in drone technology.
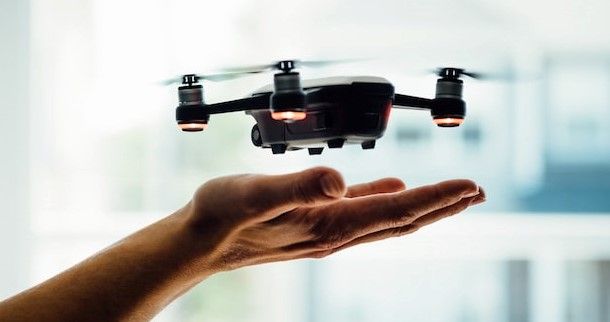
However, minute flaws may result in sparks that burn the elastomer and ruin the device. To resolve this issue, about 15 years ago researchers developed a method called ‘self-clearing’ to stop DEA failures caused by a single microscopic flaw. In this procedure, a high voltage is applied to the DEA to isolate the local electrode around a tiny defect. With the rest of the electrode disconnected from the damage the artificial muscle continues to function.
Key to this breakthrough was the application of super-strong carbon nanotubes in an optimal concentration. By having fewer carbon nanotubes in the electrode, the team was able to improve the self-clearing process as higher temperatures were reached more easily, quickly burning away the damaged electrode.
As drone designers know, there is always a balancing of assigning power for what the drone needs to do against assigning power to maintain flight and control.
“At a certain point, you will not be able to get enough energy out of the system, but we need a lot of energy and power to fly the robot,” says Chen. “We had to find the optimal point between these two constraints — optimize the self-clearing property under the constraint that we still want the robot to fly.”
To recover from major damage, such as a large hole in the wing, the MIT team used lasers to cut along the outer ridge of any defect. The self-clearing process then burnt off the damaged electrode to isolate the fault.
“In a way, we are trying to do surgery on muscles,” says Chen. “But if we don’t use enough power, then we can’t do enough damage to isolate the defect. On the other hand, if we use too much power, the laser will cause severe damage to the actuator that won’t be clearable.”
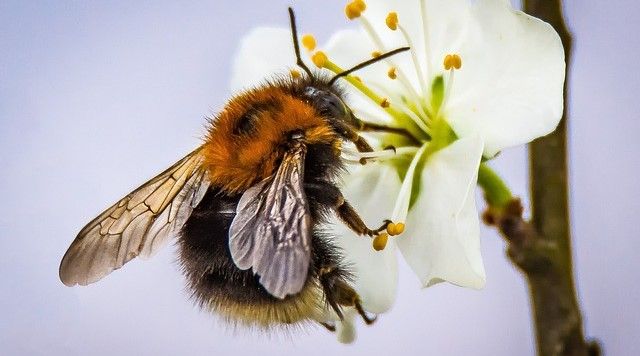
Seeing if the electrode has been separated was extremely difficult, but the team adopted earlier research which incorporated electroluminescent particles into the actuator – much like a firefly lights up. If the light was shining, they knew that the electrode was still attached, any dark patches were evidence of a successful isolation.
To test the effectiveness of this approach, the researchers poked holes in the wings with needles and burnt patches in others before measuring their ability to fly.
“Even with damaged DEAs, the repair techniques enabled the robot to maintain its flight performance, with altitude, position, and attitude errors that deviated only very slightly from those of an undamaged robot,” states the MIT report. “With laser surgery, a DEA that would have been broken beyond repair was able to recover 87 percent of its performance.”
“This work is important because small flying robots — and flying insects! — are constantly colliding with their environment,” says Nick Gravish, an associate professor in the Department of Mechanical and Aerospace Engineering at the University of California at San Diego, who was not involved with the research.
If micro drones and drone swarms are to be an effective part of the drone industry, then resolving the issue of resilience will be key - as will ease of repair.
This novel approach of combining artificial muscles, dielectric elastomer actuators, a self-clearing process, and carbon nanotubes may well show the future for a more reliable and sturdier way to fly.
Photo credit: Courtesy of the researchers at MIT, Tony Cordardo, Myriams fotos from Pixabay, Sven Lachmann, Thomas Ehrhardt, & Dose Media on Unsplash